1. Introduction
Neuromuscular balance is a key factor in improving movement efficiency, enhancing athletic performance, and reducing the risk of musculoskeletal injuries. However, it operates alongside other important contributors, such as biomechanical alignment, proprioceptive input, and environmental conditions (1, 2). Proper coordination between agonist and antagonist muscle groups ensures efficient force production by maintaining joint stability and balanced muscular activity (3). Resistance training—particularly when designed with progressive load increases—leads to various neuromuscular adaptations, including enhanced motor unit recruitment, faster firing rates (rate coding), improved proprioceptive function, and better intermuscular coordination (4, 5). These adaptations are essential for optimizing movement patterns and the functional capacity of the neuromuscular system (6).
Progressive resistance training (PRT) is well-established as an effective approach to developing strength and improving neuromuscular coordination, especially in complex, multi-joint movements performed under closed-chain conditions (7). The ability of the central nervous system to adaptively modulate recruitment strategies in response to increasing loads is vital for maintaining symmetry in muscle activation between limbs (8). Nevertheless, the extent to which progressive loading influences bilateral muscle activation remains uncertain. Some studies have observed persistent imbalances between limbs despite following structured resistance training protocols (9). This study investigates the biomechanical effects of progressive resistance training on neuromuscular balance in the upper limbs. By analyzing electromyographic (EMG) activity of agonist and antagonist muscles during closed-chain exercises, the study aims to determine how load progression affects muscle coordination and bilateral symmetry. This research aligns with recent trends in sports biomechanics that emphasize quantitative analysis to enhance understanding of performance mechanisms and optimize training outcomes (10, 11). Addressing this question could yield important insights for both performance enhancement and rehabilitation strategies.
2. Methods
This study included 22 healthy male participants aged 23–26 years, all with prior experience in resistance training. Participants were recruited from local training centers to ensure diversity in training backgrounds. Written informed consent was obtained from all individuals. The study protocol was approved by the Institutional Review Board and conducted in accordance with the Declaration of Helsinki (12). The study assessed neuromuscular balance by measuring bilateral muscle activation during closed-chain resistance exercises performed on a multi-gym machine. Each participant completed 12 sessions over three weeks (four sessions per week), with standardized warm-up, exercise, and cool-down phases. During the main exercise phase, participants performed standing elbow flexion and extension using fixed hand positions. Three intensity levels were tested in each session:
• 50% 1RM (light effort)
• 75% 1RM (moderate effort, based on expert recommendations)
• 90% 1RM (near-maximal, without inducing failure)
Each load level consisted of one set of six repetitions, executed in ascending order (50% → 90%) with 60-second rest intervals to minimize fatigue effects. This progressive structure allowed for observation of activation patterns across load intensities while maintaining safety and consistency. Muscle activity was recorded using a 4-channel surface EMG system (Delsys Trigno, Delsys Inc., USA). Bipolar electrodes were placed on the biceps and triceps brachii of both arms, following Noraxon placement standards (13). The biceps served as the agonist during flexion and the triceps during extension. Electrode placement was standardized by the same experienced researcher using anatomical landmarks. Signals were sampled at 1000 Hz, filtered (20–500 Hz), and processed to compute root mean square (RMS) amplitudes. EMG values were normalized to each participant’s maximum voluntary isometric contraction (MVIC), obtained during maximal effort against immovable resistance (13). This normalization enabled meaningful inter-limb and inter-subject comparisons. Fatigue was controlled by monitoring participant form and adherence to rest intervals. All testing conditions (time, equipment, environment) were kept consistent to reduce variability.
Data were analyzed using SPSS v24 (IBM Corp., Armonk, NY). Paired t-tests compared right and left limb activation at each intensity to assess symmetry. One-way repeated-measures ANOVA evaluated differences in EMG amplitudes across load levels. Where significant, Bonferroni-adjusted post-hoc tests identified specific differences. Significance was set at α = 0.05. Though no a priori power analysis was conducted, effect sizes (η²) were calculated to interpret the practical relevance of findings beyond p-values. The data met assumptions for normality and sphericity; Greenhouse-Geisser corrections were applied as needed (15).
3. Results
The progressive loading protocol produced a clear trend of increasing EMG activity in both agonist and antagonist muscles as intensity rose from 50% to 90% 1RM. Table 1 summarizes the peak EMG RMS values (in microvolts, μV) for each muscle group at the three tested intensities. Both the right (dominant) and left (non-dominant) sides exhibited higher EMG amplitudes at 75% 1RM compared to 50% 1RM, and a further slight increase or plateau at 90% 1RM. Notably, the values for the biceps brachii (agonist during flexion) were substantially higher than those for the triceps (antagonist during flexion) at all intensities, reflecting the prime mover versus stabilizer roles. However, by 90% 1RM the bilateral values for each muscle were very close, suggesting an attainment of neuromuscular symmetry at near-maximal effort.
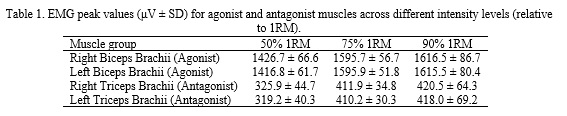
As shown in Table 1, the biceps brachii on both sides increased EMG output markedly from 50% to 75% 1RM (approximately +12% on average), with a smaller increment from 75% to 90% 1RM. The triceps brachii (acting as antagonists during the elbow flexion movement) also showed a rise in EMG as loads increased (approximately +26% from 50% to 75% 1RM), reflecting greater co-activation to stabilize the elbow joint under heavier resistance. By the 90% 1RM load, the difference in EMG between the right and left sides for each muscle was minimal (e.g., ~1 μV for biceps, ~2–3 μV for triceps, well within the SD), indicating nearly symmetric activation levels in the two arms at the highest intensity. This bilateral convergence at 90% 1RM suggests that at near-maximal effort, the neuromuscular system recruits motor units in a balanced manner between limbs.
Statistical analysis confirmed the observed trends. Paired t-tests revealed significant increases (p < 0.05) in EMG amplitude for all muscles from 50% to 75% 1RM. The further increases from 75% to 90% 1RM were smaller in magnitude and did not reach statistical significance for some muscles (in particular, the difference between 75% and 90% for biceps was not significant, indicating a plateau). Importantly, comparisons between the right and left sides at each intensity showed no significant differences at 75% or 90% 1RM (p > 0.05), corroborating that by moderate to high intensities the bilateral muscle activation was essentially even. At 50% 1RM, there were mild side differences (with the dominant side tending toward slightly higher activation), but these were not statistically significant either. Collectively, these results suggest that training load increments primarily affect the overall level of muscle activation and less so the side-to-side balance, especially at higher loads.
To further analyze the effect of load, a one-way repeated-measures ANOVA was conducted for each muscle. The ANOVA results (Table 2) indicate that load intensity had a significant effect on EMG amplitude for all muscle groups (p < 0.001). The effect sizes (η²) were large for each muscle, ranging from 0.66 to 0.82, underscoring the strong practical impact of load on neuromuscular activation. Post-hoc tests confirmed that 75% 1RM elicited significantly greater EMG than 50% 1RM in all muscles (p < 0.01 for each comparison). However, the increase from 75% to 90% 1RM was not significant for some muscles (consistent with the observed plateau). In particular, for both biceps brachii and triceps brachii on each side, the 90% vs 75% comparison yielded p > 0.05, suggesting that muscle activation may have approached a ceiling by 75% 1RM under these conditions.

Although p-values indicated strong statistical significance across the board, the η² values confirm a substantial practical impact of progressive loading on muscle activation. Interestingly, the lack of significant change from 75% to 90% 1RM in several cases (despite the numerical rise) suggests a plateau in neuromuscular activation as the load nears maximal. This plateau phenomenon, observed for both agonist and antagonist muscles, implies that by ~75% of 1RM many motor units are already recruited and additional load produces diminishing increases in EMG. Consequently, the neuromuscular system may achieve a state of near-full activation slightly before reaching true 1RM, perhaps due to limitations in additional motor unit recruitment or firing rate at that intensity. The results also showed that at the highest intensity, differences between dominant and non-dominant limbs were negligible and not significant, indicating that high-intensity closed-chain exercise can promote balanced bilateral recruitment. The antagonist muscles (triceps) displayed EMG patterns paralleling those of the agonists (biceps), with increasing activation at higher loads though at lower absolute values. This increased antagonist co-activation with heavier loads highlights its role in maintaining joint stability and controlled movement under resistance. Overall, the results support the hypothesis that progressive resistance training enhances neuromuscular coordination and promotes bilateral symmetry in muscle activation as the body adapts to higher loads.
4. Conclusion
This study examined neuromuscular responses in the upper limbs during progressive closed-chain resistance training. The findings showed significant increases in EMG activity in both the agonist (biceps) and antagonist (triceps) muscles as load increased from 50% to 90% 1RM, indicating enhanced motor unit recruitment and neural drive with rising intensity (5, 7). These increases in RMS EMG suggest that the neuromuscular system adapts by activating more motor units and increasing firing rates to meet the demands of heavier resistance, a well-established outcome of strength training (6). The consistent rise in triceps activation, despite its antagonistic role during flexion, reflects the importance of co-contraction for joint stabilization. This pattern supports previous findings indicating that higher intensities improve both intra- and intermuscular coordination, essential for maintaining joint integrity under load (6). Notably, the bilateral EMG values at 90% 1RM were nearly identical, revealing a high degree of neuromuscular symmetry between limbs. This mirrors earlier work on proprioceptive training and bilateral stability in athletic populations (1, 10, 11). A particularly interesting finding was the plateau in EMG increases from 75% to 90% 1RM. Although the load rose, the relative gain in EMG was minimal or statistically insignificant in some muscles. This suggests a ceiling effect, where most fast-twitch motor units have already been recruited, and further neural input results in limited EMG change. This concept aligns with Aagaard’s observations regarding saturation of neural drive at near-maximal efforts (5). Antagonist involvement also increased with load, but remained lower in magnitude compared to agonist activity. Nevertheless, this co-activation plays a crucial role in controlling joint motion and decelerating limb movement. Too little antagonist activation could jeopardize joint safety, while excessive activation could reduce net force output. The triceps’ balanced EMG rise suggests that participants subconsciously adjusted toward an optimal level of stabilization. This supports proprioceptive feedback models where sensory input regulates muscle activity to maintain joint integrity, especially under high loads or fatigue (2).
From a practical perspective, these findings have strong implications for training and rehabilitation. Progressive closed-chain resistance training not only enhances strength but also encourages symmetrical muscle activation between limbs. For athletes, such training may improve bilateral movement coordination in tasks like barbell lifting or swimming. In clinical settings, progressive loading may help restore neuromuscular symmetry in patients recovering from unilateral injuries, as the body seems to self-regulate activation evenly at high loads—provided the progression is safe and individualized. It is important, however, to consider inter-individual variability. Training history, muscle fiber composition, neural efficiency, and fatigue tolerance can influence adaptation speed and symmetry outcomes (3, 7). Coaches and therapists should monitor these factors when applying progressive loading strategies. Despite the promising results, some limitations should be acknowledged. The study involved only healthy young males, which limits generalizability to other populations, such as women, older adults, or clinical groups. Future studies should include more diverse samples to examine how demographic factors affect neuromuscular symmetry under load. Additionally, the use of only three intensity levels and a relatively short training protocol leaves room for future exploration. Research could investigate smaller load increments, true 1RM efforts, or EMG responses under fatigue (e.g., sets to failure). It would also be valuable to explore the impact of rest intervals and repetition schemes on symmetry and coordination. This study enhances our understanding of how progressive loading affects neuromuscular activation and symmetry in upper-limb resistance training. Under closed-chain conditions, increasing load from moderate to near-maximal levels significantly boosted EMG activity in both agonist and antagonist muscles. At 90% 1RM, muscle activation between limbs was nearly symmetrical, supporting the use of bilateral, progressively loaded exercises in sports and rehabilitation. Such training enhances motor unit recruitment, joint stability, and coordinated movement patterns. For practitioners, structured load progression offers a valuable strategy to reduce side-to-side imbalances and promote neuromuscular efficiency. In rehabilitation, careful application of these principles can help re-establish symmetry in patients recovering from unilateral impairments.
Ethical Considerations
Compliance with ethical guidelines
The research procedures strictly adhered to the ethical standards established by the institutional and national research committees, as well as international declarations concerning human experimentation. All protocols were implemented in line with the ethical principles for conducting research with human participants.
Funding
This research did not receive any financial support from government, private, or non-profit organizations.
Authors' contributions
Haider Hashim: Conceptualization, methodology design, data collection, and draft preparation; Safaa A. Ismaeel: Supervision, statistical analysis, writing-review and editing; Bashar Mohammed Ali: Literature review, EMG setup, and data interpretation; Saif Ali: Participant coordination, methodological support, and revision; Mohammed Nasir: Final manuscript formatting, referencing, and proofreading.
Conflicts of interest
The authors declare that there are no conflicts of interest associated with this article.